Vascular Biology
Vascular Diseases
This research area focuses on uncovering the signaling nodes that promote vascular growth and homeostasis. The team combines high-resolution imaging modalities, both in vitro and in vivo models of the vasculature, and traditional molecular biology techniques to ascertain features of blood vessel function in both healthy and disease states. Broadly, the research team investigates the complex role of multiple signaling pathways in the progression of vascular diseases such as atherosclerosis, vascular calcification, and arteriovenous malformations. Combining live-cell imaging with in vitro models of variable flow, the team studies how blood flow forces instruct mechanosensitive signaling pathways that control vessel function, vascular inflammation, and atherosclerosis development. Using advanced sensor technologies and laser light-sheet imaging, the team studies modes of cardiovascular development, injury, and repair in vivo. In addition, the team characterizes novel vascular repair mechanisms and pathways that mediate nitric oxide-dependent cardiovascular protection.
The team boasts advanced quantitative techniques including fused PET-CT imaging, computer-controlled biomechanical testing, and atomic force microscopy to test the effects of hyperlipidemia, exercise, and lipid-lowering agents on vascular calcification. Finally, with the addition of various disease-targeting models, our team identifies compounds that alter vascular calcification thus allowing for potential therapeutic translation.
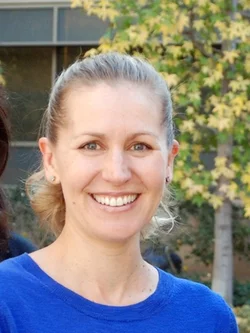
Vascular Mechanobiology
The Mack Lab studies endothelial mechanobiology in the context of cardiovascular health and disease. Specifically, the lab investigates how blood flow forces influence vascular response—both at the single cell and tissue level. To visualize how mechanical forces affect vascular function, the lab utilizes high-resolution live-cell imaging and atomic force microscopy to quantify the dynamics of endothelial cell signaling, measure flow-induced plasma membrane properties, and determine the localization of proteins at the subcellular level. By studying the mechanobiology of endothelial cell response, the lab uncovers signaling nodes that promote cardiovascular health.
eNOS: A Jekyll-and-Hyde Molecule
One explanation for variability in vascular biology may be linked to nitric oxide, long a topic of research at UCLA. In fact, Dr. Louis Ignarro, now professor emeritus in molecular and medical pharmacology, received the Nobel Prize in Physiology/Medicine in 1998 for his work demonstrating the signaling properties of nitric oxide (NO).
A question facing cardiology experts is whether an enzyme, endothelial nitric oxide synthase (eNOS), plays a positive or negative role in regulating the innermost lining of blood vessels, says Dr. Linda Cai, professor of anesthesiology and medicine (cardiology) and director of the Department of Anesthesiology’s Translational Research Program.
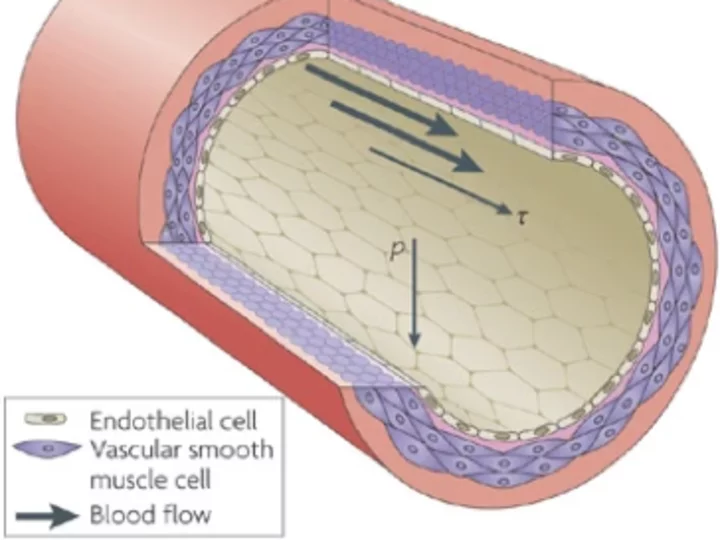
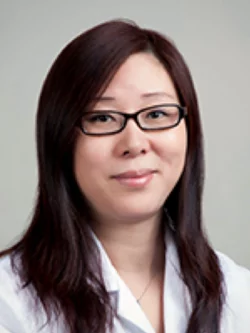
Dr. Cai’s team has made several discoveries about eNOS:
- How eNOS works in healthy conditions: When eNOS is healthy, it produces endothelial NO to dilate blood vessels and inhibit pathological events. It is a very powerful protector of vascular health because it protects the integrity of the vessels.
- When eNOS becomes dysfunctional: Dr. Cai and her colleagues found that eNOS can become dysfunctional because of normal aging, smoking, or conditions such as hypercholesterolemia. In these cases:
- It produces reactive oxygen species—toxic small molecules—that can cause significant damage to the vessel.
- Loss of healthy eNOS leads to high blood pressure, aortic aneurysms, and atherosclerotic vascular disease.
- eNOS becomes a pro-oxidant rather than an antioxidant enzyme. These reactive oxygen species play a large role in all kinds of heart and vessel disease.
- Healthy eNOS can be restored: Investigators have discovered that in animals, healthy eNOS can be restored via a newly identified pathway. This pathway uses folic acid, a B vitamin found in many fresh fruits and vegetables, especially uncooked green leafy vegetables. Folic acid helps synthesize and repair DNA.
Dr. Cai and colleagues are now conducting human studies to reveal underlying mechanisms of eNOS dysfunction and to develop new therapies in a preclinical setting.
Vascular Disease and Inflammation
Many common diseases involve chronic inflammation, but in vascular disease, inflammation is key.
Dr. Jake Lusis, who studies the complex genetic traits underlying cardiovascular and metabolic disorders, lays out the connection:
- Cholesterol accumulates in arteries.
- Inflammatory cells enter the vessel wall and produce a chronic inflammatory response.
- This response leads to a lesion.
- When this lesion ruptures, a heart attack can result.
The Red Meat-Heart Disease Connection
UCLA researchers are studying how common foodstuffs harm blood vessels. This work is part of our efforts to expand the understanding of the interactions between nature and nurture – genes and the environment.
Dr. Lusis, in collaboration with investigators from Cleveland Clinic, has discovered how the gut microbiome can cause inflammation in the heart. Articles published in Cell in December 2015 and in the Journal of the American Heart Association in February 2016 show:
- The toxic molecule, trimethylamine N-oxide, is derived through the action of gut bacteria.
- Investigators traced TMAO back to consumption of red meat.
- TMAO activates cells in blood vessels in the heart, producing inflammation.
- A naturally occurring, non-toxic molecule can inhibit the production of TMAO in gut bacteria.
This research is just the beginning of learning how gut bacteria can protect the heart.
Cholesterol-Lowering Fruit
Another group of scientists has demonstrated how tweaking another food—actually a fruit—can improve a mouse’s cholesterol profile.
Researchers at UCLA conducted experiments in mice bred to develop inflammation and atherosclerosis. In these experiments:
- Scientists inserted genes into tomatoes so that they produce a peptide, 6F, which mimics the actions of apoA-1, the main protein in high-density lipoprotein (HDL), also called good cholesterol. HDL helps mitigate harmful effects on blood vessels from low-density lipoprotein (LDL), the bad cholesterol.
- The peptide altered intestinal lipid metabolism.
- These effects in turn significantly lowered levels of inflammation and plaque formation in the arteries
According to Dr. Alan Fogelman, chair of Medicine and director of the Atherosclerosis Research Unit at the David Geffen School of Medicine, researchers are still trying to determine exactly how the peptide functions in animals, so don’t expect HDL tomatoes in the supermarket anytime soon. Still, the study is the first report in which a peptide has been engineered into food to reduce plaques and inflammation in the blood vessels of those who eat it.
Consequences of “Hardened” Arteries
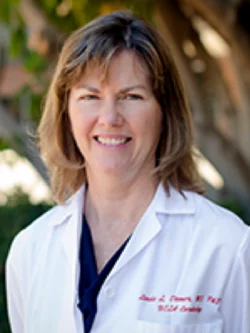
UCLA research has helped to identify the process that underlies “hardening” of blood vessels.
It has long been known that bone tissue and even marrow can form in the walls of human arteries, usually near cholesterol deposits.
Previously, says Dr. Linda Demer, vice chair of Medicine, artery wall calcification was considered a passive degenerative process. Now, work in Demer’s lab and in others indicates that artery wall cells actively form calcium deposits in much the same way that skeletal bone cells form mineral.
Together with Dr. Yin Tintut, Dr. Demer directs the Cardiovascular Biomineralization Research Group. In collaboration with UCLA engineers, they found:
- The process of calcification in the artery wall is similar to embryonic bone formation.
- There is increased risk of rupture along the edges of calcium deposits facing mechanical stress.
- By isolating and cloning the artery wall cells responsible for producing calcium mineral and by identifying the regulatory molecules controlling the process by which these cells differentiate into bone-like cells, investigators developed the first cell-based model of vascular calcification.
- Based on comparisons to cells from the skeleton, high cholesterol may contribute not only to atherosclerotic calcification, but also to osteoporosis.
Whether calcium deposits protect against, or increase the likelihood of, rupture of coronary plaques—and resulting heart attacks—is a question debated in the cardiovascular disease research community. This research is essential because of the widespread prevalence of vascular calcification, which promotes heart failure and hypertension.
Plaque: Up Close and Active
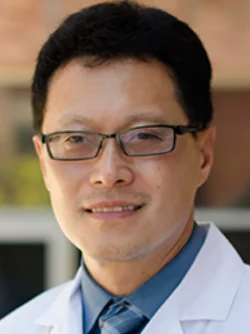
While Dr. Demer has used culture models to study plaque, the engineering side of cardiologist Dr. Tzung Hsiai, professor of bioengineering and medicine (Cardiology), favors a more direct approach. He wants to get into the heart and “look around” to identify the risks associated with plaque and blood clots.
Dr. Hsiai (pictured at right) and his group have developed advanced imaging technology and sensors to elucidate vascular mechanotransduction underlying tissue development, injury, and repair. By developing fundamental and precise optical instruments, including a hybrid of laser light-field and light-sheet, they have demonstrated hemodynamic shear stress to modulate the initiation of endocardial trabeculation, endothelial to mesenchymal transition in the outflow track, and microvascular repair following tail amputation in the zebrafish model of tissue regeneration. By microfabricating arrays of flexible bioelectronics, the lab has established intravascular deployment of wireless and leadless micropacing in the Yucatan mini-pig hearts, electrical impedance mapping of carotid endolumen laden with oxidized lipid in the mini-pigs, and electrical tomography to diagnose fatty liver infiltrates in human subjects with BMI>25 kg/m2.
By imaging processing and deep learning, they have computed 3-D microvascular network in the mouse retinal model of injury and repair, virtual reality for pre-surgical planning, and simulation of cardiac trabeculation for an interactive experience. The highly collaborative and interdisciplinary research has been inspired and enriched by a team science approach across partnering institutions, including Caltech, Cornell, Columbia, Mayo Clinic, UCSD, USC, Stanford University.