Generating and Regenerating Neural Circuits
The Developing Nervous System
Understanding the Mechanisms
The extraordinarily diverse functions of the nervous system, from cognition to movement, are possible because neurons are assembled into precisely ordered networks that permit them to rapidly and accurately communicate with their synaptic targets. The Butler laboratory seeks to understand the mechanisms that establish these neuronal networks during development with the long-term goal of determining how this process may be co-opted to regenerate diseased or damaged circuits.
Our studies have revealed there are also “temporal” guidance signals, which regulate the rate at which axons grow towards their targets thereby permitting neural circuits to develop in concert with the rest of the developing embryo.
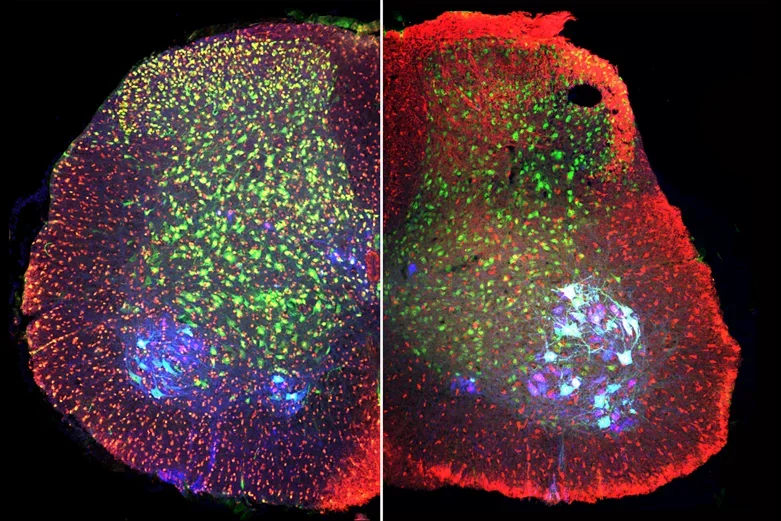
Postnatal mouse spinal cord labeled with antibodies against phospho-cofilin1 (red, left), cofilin (red, right) ChAT (blue, motor neurons) and NeuN (green, all neurons) shows that the levels of both total and inactive cofilin1 remain high in postnatal development.
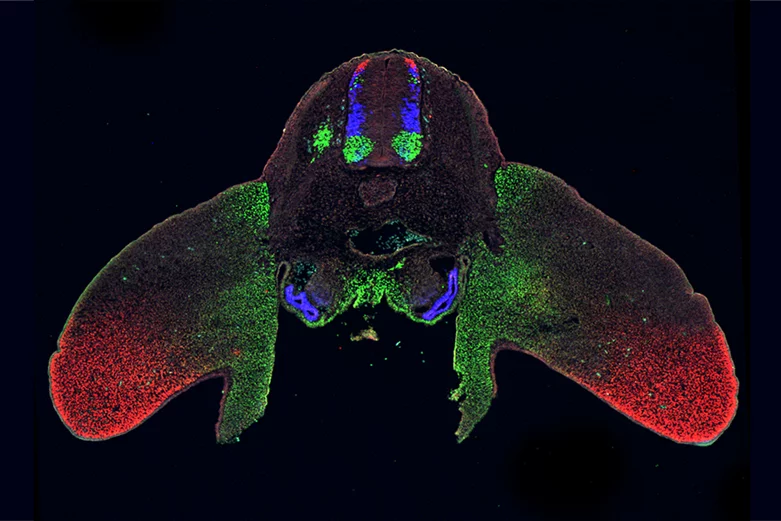
Transverse section through a day 4 chicken embryo labeled with antibodies against Lhx2 (red), Lhx3 (blue) and Isl1 (green), shows how the same set of transcription factors are used to specify cellular identity in multiple places in development, here the spinal cord, limb and kidneys.
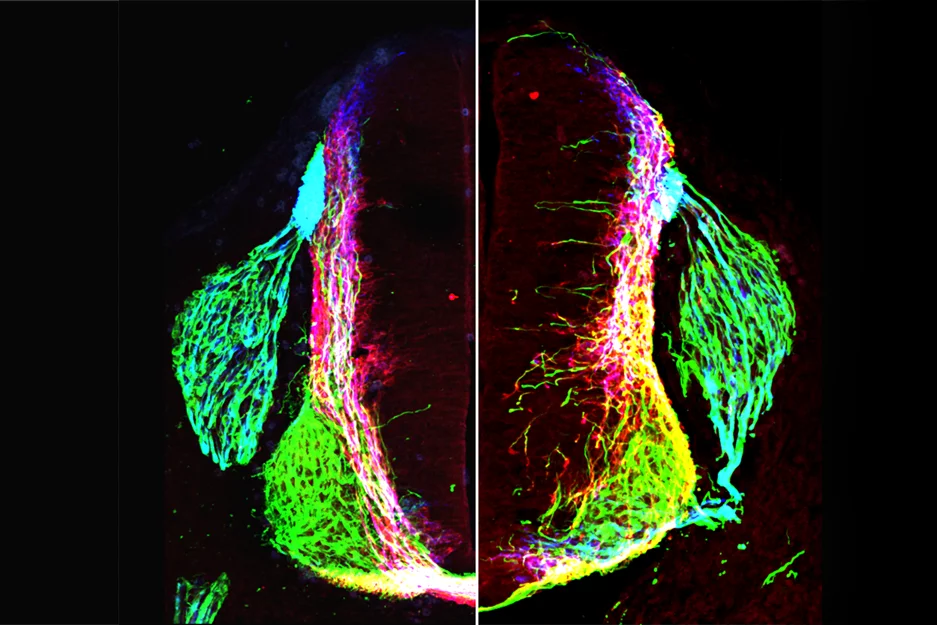
Transverse section of control (right) or netrin1 (mutant) mouse embryonic spinal cord showing axons labeled with antibodies against neurofilament (green), Robo3 (red) and Tag1 (blue).
Bone Morphogenetic Proteins (BMPs) establish sensory circuitry in the spinal cord
The Butler laboratory has a long-standing interest understanding how a single class of molecular signals directs distinct cellular consequences during neuronal circuit formation. Working in the developing spinal cord, they have shown that Bone Morphogenetic Protein (BMP) family of growth factors has signal specific roles directing the identity of sensory interneurons, the neurons that permit us to experience and react to the environment. Once formed, classes of sensory interneurons then use the BMPs to direct their early axonal extensions. Butler and her colleagues are now determining the key intrinsic mechanism(s) that translate the ability of the BMPs to sequentially direct cell fate and axon guidance decisions, two strikingly different processes in the generation of neural circuits. This problem is largely unresolved in developmental biology and may be of fundamental to understanding how best to deploy growth factors to regenerate neural circuitry damaged by injury or disease.
Identification of temporal guidance mechanisms during neural circuit formation
Work in the axon guidance field has focused on identifying the directional signals that orient axons spatially. However, the embryonic environment is constantly changing, making it critical that axons reach such directional cues at a particular time during development. Butler's studies have revealed there are also “temporal” guidance signals, which regulate the rate at which axons grow towards their targets thereby permitting neural circuits to develop in concert with the rest of the developing embryo. The Butler laboratory is currently assessing how this mechanism can be harnessed to accelerate the regeneration of injured peripheral nerves. The successful implementation of this technology could result in significantly improved recovery times for patients with damaged nervous systems.
Reinterpreting the role of netrin1 in the spinal cord
Axons navigate to their destinations using guidance cues in the embryonic environment to direct the path of axon extension. Axons have long been thought to be guided by chemotropic guidance cues: long-distance diffusible signals that function like a harbor beacon orienting a ship from afar. The textbook example of this mechanism was the ability of netrin1 to guide commissural axons in the developing spinal cord. However, recent studies conducted by Butler and her colleagues have shown that netrin1 promotes axon extension by haptotaxis, not chemotaxis, i.e. the directed growth of cells along an adhesive surface, akin to the holds used by climbers to pioneer their way to the top of a mountain. They have shown that the bipolar geometry of neural progenitor cells permits them to establish a local netrin1+ substrate along basal edge of the spinal cord. This netrin1+ substrate then positions and promotes axon outgrowth, so that axons grow in a specific direction. These studies show that neural progenitors cells have an intrinsic capacity to direct axon extension, opening new avenues by which netrin1 could be deployed to direct regenerative axonal growth.