Mitochondria DNA
The Connection to Neurological Diseases
What Is Mitochondria DNA?
Neurodegenerative diseases such as Alzheimer’s disease (AD), Parkinson’s disease (PD), Lou Gehrig’s disease (ALS or amyotrophic lateral sclerosis), and frontotemporal dementia (FTD) cause enormous human suffering, strain families, and pose large challenges for societies and healthcare systems. None of these diseases has a cure. AD and PD together afflict half of the population over the age of 80. While deaths due to cancer, heart disease, stroke and HIV have declined since 2000, deaths due to AD have increased by 70 percent. The reason we do not have effective prevention or treatment is because we do not understand the fundamental biology of neurodegeneration.
Mitochondria DNA Testing
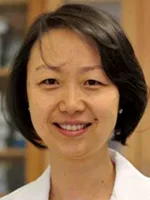
Dr. Ming Guo, a UCLA scientist and board-certified practicing neurologist, is at the forefront of discovering why cells in the brain degenerate and how the process can be stopped. Dr. Guo’s lab studies neurodegenerative diseases (including AD, PD, FTD, and myopathy) and regeneration. She uses models of these diseases and patient cells to identify the underlying causes of neurodegeneration in order to develop effective treatments. Much of her work focuses on the role of mitochondria—small organelles that are present in every cell—in aging and disease. Mitochondria generate the bulk of the energy used to power the brain, muscle, heart, and other tissues. Each mitochondrion has a genome. Unfortunately, mitochondria DNA naturally accumulates damage as we age. Damage to mitochondria DNA can lead to decreased energy production and cell malfunction, which promote age-related diseases such as AD and PD, diabetes, cancer, heart disease, and loss of muscle strength. Dr. Guo has identified key genes that promote healthy mitochondrial function and have the ability to eliminate defective mitochondria DNA. These discoveries enable the development of therapies that prevent neurodegeneration.
Mitochondria DNA News & Research
- Dr. Guo and her team made a landmark discovery in the field of Parkinson’s disease. They discovered that two PD genes, PINK1 and parkin, promote mitochondrial health and survival of neurons and muscle. Cells use this pathway to sense and respond to mitochondria DNA damage, repairing or removing damaged mitochondria DNA, a form of quality control that maintains cell health over our lifespan.
- The team has identified several molecules that reverse the cell damage caused by defects in PINK1/parkin that cause PD in disease models.
- Dr. Guo has discovered that activation of the PINK1/parkin pathway, as well as several other treatments, can cause the removal of defective mitochondria DNA from adult cells in disease models. This finding means that a key feature of aging can be therapeutically reversed.
- Dr. Guo and her team have found that drugs that improve mitochondrial health can stop FTD and myopathy in disease models.
- Researchers identify potential treatment for type of muscle and brain degenerative disease
Mitochondria Is The Powerhouse Of The Cell
Why Are Mitochondria Called The Powerhouse Of The Cell?
Choice and preference help to shape everyday life for many of us, and what we eat to fuel our bodies is certainly no exception. Preferring one type of food to another, informing a decision as simple as restaurant choice or selection off a menu, can have repercussions lasting far longer than the few hours it takes to digest the meal. Interestingly, this concept of fuel preference manifests itself at the cellular level as well. Mitochondria are the power plants of our cells, and these power plants can churn through sugar metabolites, amino acids, and fatty acids to harvest biological energy. It turns out that it’s not just how much biological energy these power plants produce, but also what fuels they’re burning that matters to our bodies.
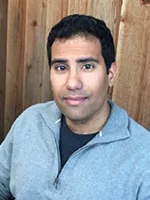
This is perhaps best exemplified by our muscle tissue. When fasting, our muscle shifts to burning fat stores and suppresses glucose (carbohydrate) metabolism. Upon breaking the fast, our muscles switch to robust glucose oxidation and shut off fatty acid breakdown. This flexibility is a hallmark of healthy muscle tissue, and disruptions in healthy fuel ‘prefereces’ underlie diseases as varied as type 2 diabetes, cardiac disease, neurodegeneration, and perhaps even certain cancers. But what if we could target this fuel preference at a cellular level? If we restore a healthy balance between the nutrients our bodies use as fuel can we combat these diseases? If those questions can be answered, and we better understand the links between metabolism to physiology, they could be part of the puzzle for major breakthroughs in human health.
Mitochondrial Metabolism
Dr. Divakaruni’s new research laboratory is focused on developing mitochondria as therapeutic targets and, specifically, targets that promote metabolic flexibility and restore healthy nutrient preference in tissues. A current focus is how fuel preference in the brain may adjust neuronal physiology. The metabolic demands of the brain punch well above their proverbial weight: although it accounts for only about 2% of our body weight, it requires roughly 20% of our body’s energy budget. The dominant and requisite fuel of the brain is glucose, but to what extent the brain can use amino acids to fuel energy metabolism may have far-reaching implications.
Glutamate, for example, is the main excitatory neurotransmitter in the brain and involved in the synaptic plasticity associated with learning and memory. Under conditions of energy stress, however, extracellular glutamate can accumulate to toxic levels that trigger a cascade of neuronal death. Recent work by Dr. Divakaruni and colleagues, however, has shown that reducing mitochondrial glucose oxidation in neurons rewires metabolism to rely on glutamate to fuel energy metabolism. This, in turn, reduces the amount of glutamate apportioned for release. Excitingly, the result establishes mitochondrial fuel preference as a therapeutic target for the many acute and chronic neurodegenerative diseases characterized by glutamate excitotoxicity.
Mitochondrial Metabolic Disease
Role Of Mitochondria In Metabolism
Passionate about developing new methods to study mitochondria and metabolism, Dr. Divakaruni’s research laboratory is excited to apply new approaches to longstanding questions about how metabolism can alter cell physiology. For example, prior to advances in fluorescence microscopy, few (if any) imagined the dynamic mitochondrial “life cycle” of fusion, fission, mitophagy (clearance of damaged mitochondria), and biogenesis (forming new mitochondria) studied by many members of the UCLA metabolism theme. In the same way, there is huge potential for recent methods and technology development to add to, or perhaps rewrite, our textbook understanding of nuts-and-bolts metabolism. For example, can the “yin-and-yang” relationship between glucose and glutamate in the brain help explain the decades-old observation that low carbohydrate, “ketogenic” diets can be effective in treating medically refractory seizure disorders? Studying such links between physiology and metabolism as our research tools continue to improve has tremendous potential for revealing new pathways to target and treat human disease.